“Photocatalysis enables us to harness the energy generated by the Sun and convert it into chemical energy stored in hydrogen molecules. The produced hydrogen gas can be used as a clean, renewable fuel source that does not emit greenhouse gases or other harmful substances. When burned or used to produce electricity, the only by-product of hydrogen is water, resulting in a clean and sustainable energy cycle.” – Dr. Alexander Bondarchuk, Technological University of Mixteca
With so many paths forward in our pursuits for cleaner energy for the future, concerns about how to store energy from intermittent renewable sources are coming more and more to the forefront of people’s minds.
As an energy vector, hydrogen is one of the most commonly touted solutions to address the pressing need for a clean and efficient fuel source to power industry, transportation, and to support a diversified electrical grid.
Current methods for producing and distributing clean hydrogen are either energy-intensive, prohibitively expensive or limited by existing infrastructure.
That is why research into novel methods to produce hydrogen utilizing solar is becoming of great interest.
The ability to produce energy in situ on location means the removal of the difficulties associated with shipping hydrogen from centralized production locations. The costs tied to the logistics of transporting and the dangers of needing to move to compressed hydrogen become a thing of the past; by switching to a solar-based production method, the environmental impact of that production by providing alternatives to current fossil fuel-based processes.
To reach the point where the sun is a viable producer of hydrogen, it all starts with scientists such as Dr. Alexander Bondarchuk researching and refining the process.
Vision of the Technological University Of Mixteca: Research to Improve and Uplift
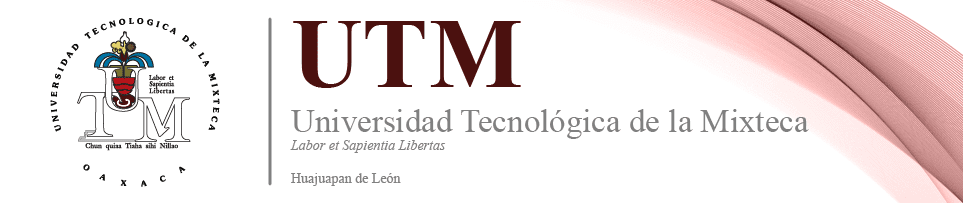
Dr. Alexander Bondarchuk is a professor at the Technological University of Mixteca in Oaxaca, Mexico. Founded just over 30 years ago, the Technological University of Mixteca was built on the dream of Dr. Modesto Seara Vázquez, who envisioned that higher education could lift not just people but an entire culture out of hardship and into prosperity.
To quote Dr. Bondarchuk:
“The successful realization of this unprecedented academic project in Mexico has resulted in the economic development of the region and the foundation of UTM”, which in 2023 stands as one of the top ranked Universities within the region.
Dr. Bondarchuk embodies the ideals of his institution with his work on developing photoelectrodes for the large-scale production of hydrogen via photocatalytic hydrogen evolution from water.
By pushing forward the boundaries of our understanding of this process, there is hope of helping to create the employment opportunities of the future, but alternatives to our current energy systems that could benefit not just Oaxaca or even Mexico but potentially hundreds of millions of people across the globe.
Currently focusing on catalytic coatings such as Hematite (∝-Fe2O3) and Bismuth Vanadite (BiVO4) on freestanding porous Tin Oxide (SnO2) based ceramic substrates, Dr. Bondarchuk hopes to create low-cost, high-performance photoelectrodes to enable large-scale hydrogen production, harnessing the reliable and almost inexhaustible power of the sun.
The Challenges
Catalytic Materials Scale-up Costs
Traditionally, catalytic materials used for hydrogen production are Platinum, Palladium or other platinum group elements that are significantly rarer and thus more expensive than gold.
Trying to scale up hydrogen production from an already substantial ~120 Million metric tonnes per year is a challenging feat, let alone shifting that amount into cleaner production methods instead of the current carbon-intensive processes we use. Using an already scarce and exorbitantly priced material is a non-starter to help turn our transport sector away from fossil fuels and provide a source of backup generation for our electrical grids.
Catalytic Materials Operational Difficulties
Add that to the other requirements of an operational photocatalyst instead of a traditional metallic catalyst, and that proposition becomes more difficult still. It means needing to be a semiconductor with an appropriate band edge position for reduction/oxidation of water (ideally, intrinsically catalytic) to split water (1.23V or more) and also able to withstand highly acidic or basic aqueous solution environments without degradation during operation. Keeping all of this in mind, Dr. Bondarchuk must find a viable material that isn’t prohibitively expensive, and it starts looking like a tall order.
Operational Efficiency of Solar to Hydrogen Production
Once a potentially viable material is found, researchers like Dr. Bondarchuk still have to qualify it to prove that it can perform at an acceptable level to compete on the market. In photocatalytic hydrogen production, this has a rough metric in the form of the Incident Photon-to-Current Efficiency (IPCE) and Solar to Hydrogen Efficiency (STHE).
IPCE measures the conversion efficiency between photons that strike the active material and the measured current from the device. This is analogous to the External Quantum Efficiency (EQE) characterization performed for photovoltaic solar cells, especially as it is also measured as a function of incident wavelengths.
In photovoltaic solar panels, Power Conversion Efficiency (PCE) measures the electrical output energy relative to the known incident light energy. Similarly, STHE measures the amount of energy produced in the form of hydrogen by the device in question when exposed to a known light intensity.
But unlike PCE, STHE is only possible to measure directly with expensive and time-consuming machinery and processes such as gas chromatography or mass spectroscopy. In general, the IPCE is commonly used as a comparable measure of efficiency instead. This is due to the ease of measuring the electrical current produced by the system when exposed to a known or controllable incident light source.
What is most important, though, is the accepted threshold for what is considered a viable photocatalytic material, generally agreed to be a 10% STHE equivalent performance. 10% might sound low compared to regular photovoltaic devices, reaching the low 30% range for efficiency. Still, the benefits of hydrogen, such as being able to be transported off-grid and used as a storage mechanism for other sources of renewable energy, means that the lower efficiency is acceptable due to the provided utility of the product.
“To the best of our knowledge, the photoelectrodes which satisfy all these requirements and would have low cost in their large-scale manufacturing needed for commercially viable hydrogen production have not yet been obtained. Existing photoelectrodes with required solar-to-hydrogen (STH) efficiency are complicated to fabricate or require expensive materials like Pt or Au, hindering large-scale production and application.” – Dr. Alexander Bondarchuk
The Solution
Low-Cost Semiconductors: Is Iron Oxide The Future Of Solar Hydrogen Production?
Of the common types of catalysts, platinum group elements are too expensive to be used on the scale needed for a burgeoning new industry. Substituted metallic catalysts such as cobalt alloys can experience problematic chemical reactions in the highly conductive electrolytes required for hydrogen production, such as oxidation most commonly, but other complexing reactions can also occur, depending on the composition of the electrolyte and other solid components in the cell.
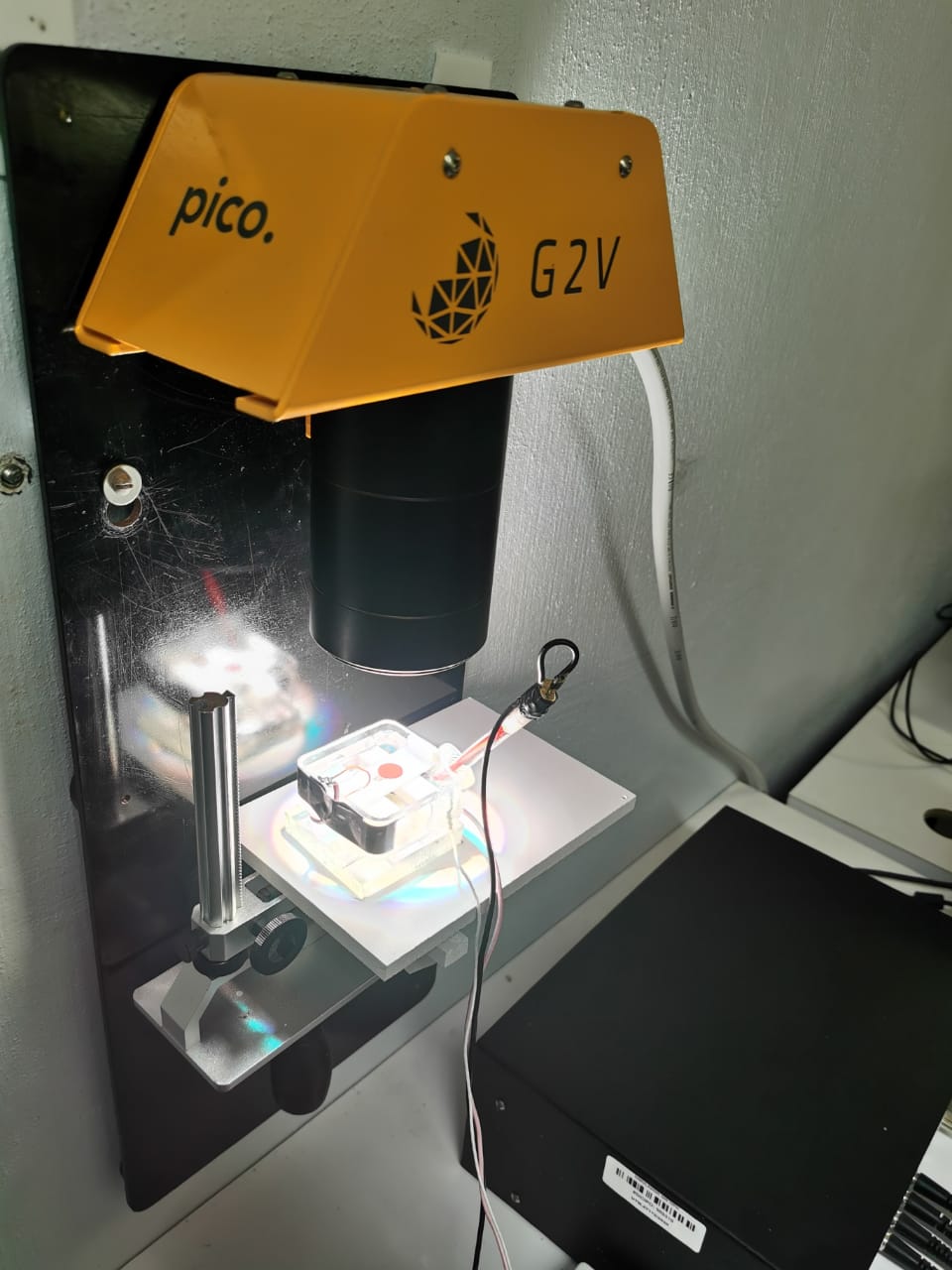
Additionally, neither of these metallic catalyst material groups possesses the semiconductive properties needed for photocatalytic water splitting. Currently, they are used to produce hydrogen in thermal or electrical processes that rely heavily on fossil fuel-based energy.
To that end, Dr. Bondarchuk and his group have focused on an unassuming material… rust. More accurately called Hematite, it is an oxide of iron that is highly stable to chemical attack and has a band-gap of 1.9 – 2.2 eV depending on the phase structure.
While rust is cheap, applying a nanometer-scale coating of acceptably pure hematite to a substrate is still quite an intensive process, and validating the application of hematite as a photoelectrode material for a full photoelectrochemical cell is enough to keep any researcher busy.
Discoveries that can change the world take time, knowledge and a scientific instrument that is as forward-thinking as the researcher.
Validating Small Scale Lab Based Hydrogen Production Performance with a Class AAA Light Source
“[The G2V Pico] is one of the principal instruments for researchers who are working in the area of solar energy materials.” – Dr. Alexander Bondarchuk on the G2V Pico
As of 2022, Dr. Bondarchuk has reported the fabrication of a hematite photoanode that produced a photocurrent density of 0.63 mA/cm^2 at 1.23V when measured against a Reversible Hydrogen Electrode (RHE). This was done using their G2V Pico to simulate an AM1.5G exposure (350 nm – 1100 nm; 79.1 mW/cm^2) on a pure hematite photoanode grown on a porous SnO2 substrate.
To improve the future performance of these half-cell devices, Dr. Bondarchuk hopes to investigate the potential for including cocatalysts within the hematite surface coating or even developing full hetero-unions of hematite and other catalytic materials to improve STH efficiency.
While there is a limit to the energies that can be usefully utilized due to the chemistry of hydrogen splitting and the band gap of the catalytic material, validating a device that can use as much of the available energy as possible is a lot easier when there are instruments such as the Pico that can support those investigations.
To support Dr. Bondarchuk’s efforts, he required control over the output spectra beyond what traditional solar simulators were capable of.
He required an instrument built in the future but available in the present.
The Future of Photochemistry Testing: Software Controllable Variability, Exceptional Stability, and The Ability to Effectively Measure Rates of Reactions
A tuneable LED solar simulator like the Pico is what Dr. Bondarchuck needed. It allows him and his team to carefully examine how different portions of the solar spectrum can influence the catalytic reaction rate.
Adjusting the wavelength and known intensity of output throughout the spectrum is an invaluable tool for optimizing the photosensitive efficiency of the catalytic materials for the entire solar spectrum.
But there is more than just efficiency to consider. He needed stability over time to conduct his research.
A material meant to operate in ambient sunlight and reliably produce hydrogen in industrial quantities has to have the reliability and longevity to make good on that promise. With the Pico, Dr. Bondarchuk can test materials under conditions representative of the 24-hour solar cycle through software automation without having to worry about his light source experiencing unexpected variations throughout the experiment.
This gives his lab an added layer of confidence in the accuracy of their experiments and repeatability.
Towards a Future Powered by Clean Energy and Clean Fuels
The goal of bettering the life of everything on this planet comes down to those willing to rise to the challenge of producing cleaner methods to power the world.
Others like Dr. Bondarchuk quietly rise to this challenge time and time again with an overarching goal – making the world better. Their important work is carried out thanks to institutions like the Technological University of Mixteca, giving them the space to unleash their expert knowledge and unparalleled problem-solving skills on issues of great concern.
This positive feedback loop between researchers and their respective institutions on tackling climate change has become apparent in more places than just Oaxaca. It is time we start praising their efforts and turning these world changers into household names.
At G2V, we are proud to assist Dr. Bondarchuk and the Technological University of Mixteca on their journey to building a foundation for a cleaner tomorrow.
References
https://www.utm.mx/profesores/olexandr_bondarchuk.html
https://www.utm.mx/index_eng.html
https://en.wikipedia.org/wiki/Modesto_Seara_V%C3%A1zquez
https://www.4icu.org/mx/oaxaca/
[1] Alexander N. Bondarchuk, Iván Corrales-Mendoza, Josué A. Aguilar-Martínez, Ulises M. García-Pérez, Frank Marken, Porous and conductive SnO2 ceramics as a promising nanostructured substrate to host photocatalytic hematite coatings: Towards low cost solar-driven water splitting, Catalysis Communications, 174(2023), 106593-8, ISSN 1566-7367, https://doi.org/10.1016/j.catcom.2022.106593.
[2] A. N. Bondarchuk, I. Corrales-Mendoza, J. A. Aguilar-Martínez, S. A. Tomás, D. A. Gómez-Caiceros, A. Hernández-Méndez, Frank Marken, BiVO4 Photoanode Grown on Porous and Conductive SnO2 Ceramics for Water Splitting Driven by Solar Energy, Ceramics International 46 (2020) 9040–9049. https://www.sciencedirect.com/science/article/abs/pii/S0272884219336429?via%3Dihub
https://www.irena.org/Energy-Transition/Technology/Hydrogen
https://www.perfectlight.com.cn/technology/detail-56.html
https://www.nature.com/articles/s41586-022-05399-1
https://g2voptics.com/photovoltaics-solar-cells/solar-cell-testing/